Disperse Phase Transition Processes
In a large number of technical processes involving droplets and particles, transition processes take place between solid, liquid and gaseous. Typical examples of such disperse phase transition processes are atomization processes with the aim of particle generation through solidification of the droplets or the local welding of particles in additive manufacturing to form parts. Gaining a fundamental understanding of these processes and transferring this knowledge to application is done on the basis of isolated sub-processes - so-called generic model experiments - and on the basis of process modeling.
Research Focus
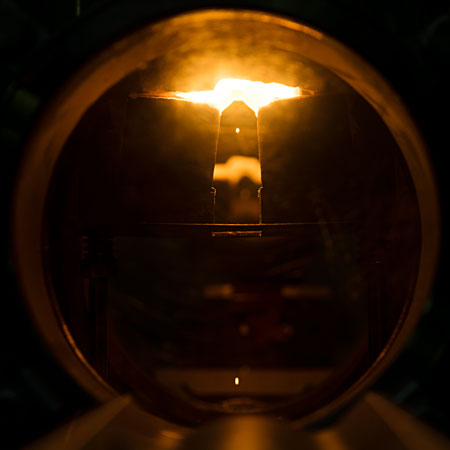
Experiments with metallic single droplets allow the reproducible adjustment of droplet sizes from approx. 200 µm in variable gas atmospheres from metallic alloys at temperatures of up to 1650 °C. On an inert drop distance of 6.5 m, the droplets either fall freely or can be quenched after various distances in a fluid or deposited on a substrate. There are many possibilities here for sample generation from a wide range of materials, e.g. to determine the microstructures or physical properties of materials at specific cooling rates.

Thermophysical material values such as surface tension or viscosity are essential for process control in processes such as atomization, welding or additive manufacturing. At the same time, however, these material values are very challenging to determine using conventional methods and are therefore only available to a limited extent and under ideal process conditions.
Quantitative analysis of droplet oscillation in free fall after droplet formation allows flexible determination of the surface tension and viscosity of a metal melt as a function of temperature, including in reactive atmospheres or atmospheres of technical purity. Furthermore, a more detailed analysis allows the simultaneous determination of the liquid density.

Processes with metallic droplets and additive manufacturing processes involve solidification or glass formation processes. These solidification processes depend on spatially resolved thermal histories which determine the morphology and its fineness. Rapid solidification occurs during the aforementioned processes, for which simple equilibrium models are often no longer applicable. The modeling of these processes on the basis of heat, momentum and mass transfer with the integration of different scale solidification models (also based on the phase field method) allows the identification of process paths for the setting of specific properties.
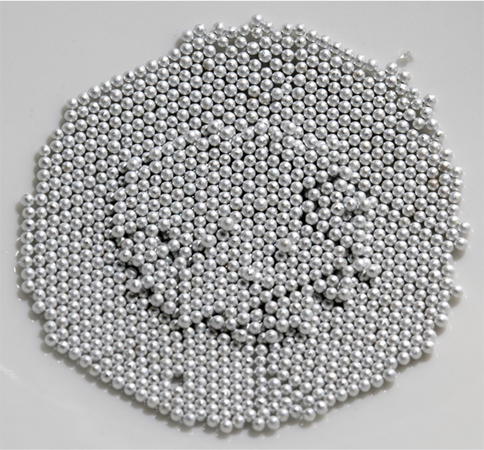
In times of climate change, strategies for energy-efficient recycling of materials play an increasingly important role. This includes strategies to compensate for contamination resulting from recycling in order to minimize the need to separate materials.
The range of existing processes from casting to droplet processes to additive manufacturing offer a variety of opportunities for strategy development on a model scale with the possibility of subsequent scale-up.
Hydrogen as an important process gas and possible energy carrier of the future places new demands on materials. The processing of metal melts in hydrogen-rich atmospheres allows for the rapid synthesis of hydrogen-loaded materials to determine, for example, embrittlement properties. At the same time, particles on the milimeter scale are used to efficiently develop new material concepts for hydrogen transport and storage on the laboratory scale.
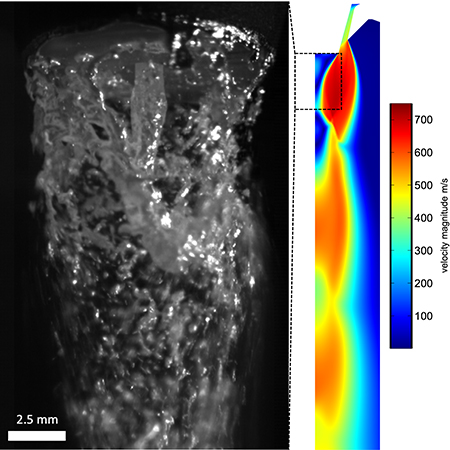
The design and application of atomization processes for molten metals has been a research topic at the University of Bremen for decades, and has become much more important with the growth in the field of additive manufacturing. The design of nozzle systems with CFD methods on the scale from the near-field to the entire spray tower allows a systemic perspective on the process. In the past, a novel convergent-divergent nozzle for close-coupled atomization has increased the stability and, at the same time, the efficiency of atomization. Developed nozzle systems are used in our spray towers in order to be able to atomize not only low-reactive melts, e.g. on Fe- or Cu-basis, but also aluminum or metallic glasses.

In addition to conventional metallic materials, several new classes of materials have become popular in recent decades, such as metallic glasses, high-entropy materials and quasicrystals. What these materials have in common is that, in addition to very specific compositions and generally low levels of contamination, they require very specific thermal histories that have to be adjusted by adapted process control of the entire process chain. In the case of metallic glasses, for example, oxygen contamination must be kept low throughout the process chain from melting to powder generation to additive manufacturing. At the same time, specific critical cooling rates must not be understepped in additive manufacturing.
Published Models

With this model, the PBF-LB/M process can be modeled. In addition to the flow and temperature field, the concentration field is also solved, so that different particle mixtures and various combinations of powder and substrate materials can also be modeled. The particle layers are applied via a DEM simulation and transferred to the model. The heat input is modeled via a ray tracing approach.
The model is implemented in OpenFoam and can be used freely.
Download from Leibniz-IWT's GitHub: github.com/Leibniz-IWT/Metal-Mixing-in-LPBF

This model is an implementation of the Ferreira et al. model for dendritic solidification of pure elements. The model is implemented in Comsol Multiphysics and can be adapted very easily to different geometries and boundary conditions.
Download in the GitHub of Leibniz-
IWT:https://github.com/Leibniz-IWT/comsol-dendrite
Selected Publications
[1] E. Gärtner, A. Witte, N. J. Peter, V. Devulapalli, N. Ellendt, G. Dehm, E. A. Jägle, V. Uhlenwinkel, L. Mädler: Melt pool signatures of TiN nanoparticle dry-coated Co25Cr25Fe25Ni25 metal powder in laser-powder-bed-fusion, Materials & Design 2023, 226, 10.1016/j.matdes.2023.111626.
[2] M. Frey, J. Wegner, E. S. Barreto, L. Ruschel, N. Neuber, B. Adam, S. S. Riegler, H.-R. Jiang, G. Witt, N. Ellendt, V. Uhlenwinkel, S. Kleszczynski, R. Busch: Laser Powder Bed Fusion of Cu-Ti-Zr-Ni Bulk Metallic Glasses in the Vit101 Alloy System, Additive Manufacturing 2023, 10.1016/j.addma.2023.103467.
[3] A. Chouhan, M. Hesselmann, A. Toenjes, L. Mädler, N. Ellendt: Numerical modelling of in-situ alloying of Al and Cu using the laser powder bed fusion process: A study on the effect of energy density and remelting on deposited track homogeneity, Additive Manufacturing 2022, 59, 10.1016/j.addma.2022.103179.
[4] N. Ellendt, B. Clausen, N. Mensching, D. Meyer, C. Plump, H. Sonnenberg, M. Steinbacher, A. Toenjes: Experimental Methods to Enable High-Throughput Characterization of New Structural Materials, Jom 2021, 73, 3347-3355 10.1007/s11837-021-04901-w.
[5] D. Beckers, N. Ellendt, U. Fritsching, V. Uhlenwinkel: Impact of process flow conditions on particle morphology in metal powder production via gas atomization, Advanced Powder Technology 2020, 31, 300-311 10.1016/j.apt.2019.10.022.
[6] S. I. Moqadam, L. Madler, N. Ellendt: Microstructure Adjustment of Spherical Micro-samples for High-Throughput Analysis Using a Drop-on-Demand Droplet Generator, Materials (Basel) 2019, 12, 10.3390/ma12223769.
[7] S. Imani Moqadam, L. Madler, N. Ellendt: A High Temperature Drop-On-Demand Droplet Generator for Metallic Melts, Micromachines (Basel) 2019, 10, 10.3390/mi10070477.
[8] N. Ellendt, A. M. Lumanglas, S. I. Moqadam, L. Mädler: A model for the drag and heat transfer of spheres in the laminar regime at high temperature differences, International Journal of Thermal Sciences 2018, 133, 98-105 10.1016/j.ijthermalsci.2018.07.009.
[9] D. Schwenck, N. Ellendt, J. Fischer-Bühner, P. Hofmann, V. Uhlenwinkel: A novel convergent–divergent annular nozzle design for close-coupled atomisation, Powder Metallurgy 2017, 60, 198-207 10.1080/00325899.2017.1291098.
[10] K. G. Prashanth, H. Shakur Shahabi, H. Attar, V. C. Srivastava, N. Ellendt, V. Uhlenwinkel, J. Eckert, S. Scudino: Production of high strength Al85Nd8Ni5Co2 alloy by selective laser melting, Additive Manufacturing 2015, 6, 1-5 10.1016/j.addma.2015.01.001.
Further publications:
Google Scholar (Link: https://scholar.google.de/citations?user=6MPB5br_W0QC&hl=en&oi=ao)
OrcID (Link: https://orcid.org/0000-0001-7242-8063)
Scopus
(Link: www.scopus.com/authid/detail.uri)
Work Group
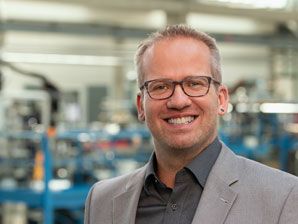
Dr.-Ing. Nils Ellendt
Head
Universität Bremen
Fachbereich 4 Produktionstechnik
Fachgebiet 01 Mechanische Verfahrenstechnik
IW1+2 1040
+49 421 64519
ellendtprotect me ?!iwt.uni-bremenprotect me ?!.de
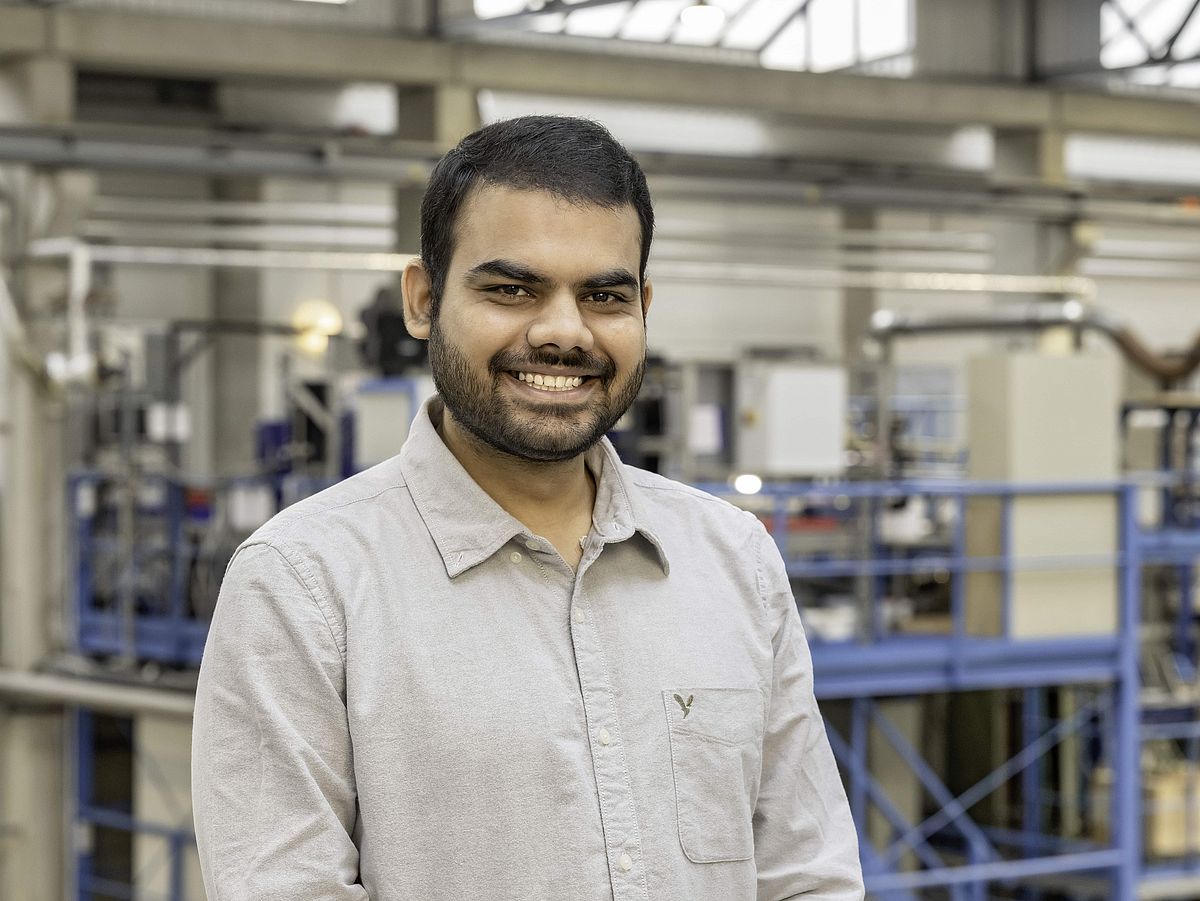
M. Tech. Arvind Chouhan
Modeling of additive manufacturing processes
Universität Bremen
Fachbereich 4 Produktionstechnik
Fachgebiet 01 Mechanische Verfahrenstechnik
IW1+2 1010
+49 421 64507
a.chouhanprotect me ?!iwt.uni-bremenprotect me ?!.de

M. Sc. Kiana Fahimi
Determination of thermophysical properties
Universität Bremen
Fachbereich 4 Produktionstechnik
Fachgebiet 01 Mechanische Verfahrenstechnik
IW1+2 1010
+49 421 64503
fahimiprotect me ?!iwt.uni-bremenprotect me ?!.de
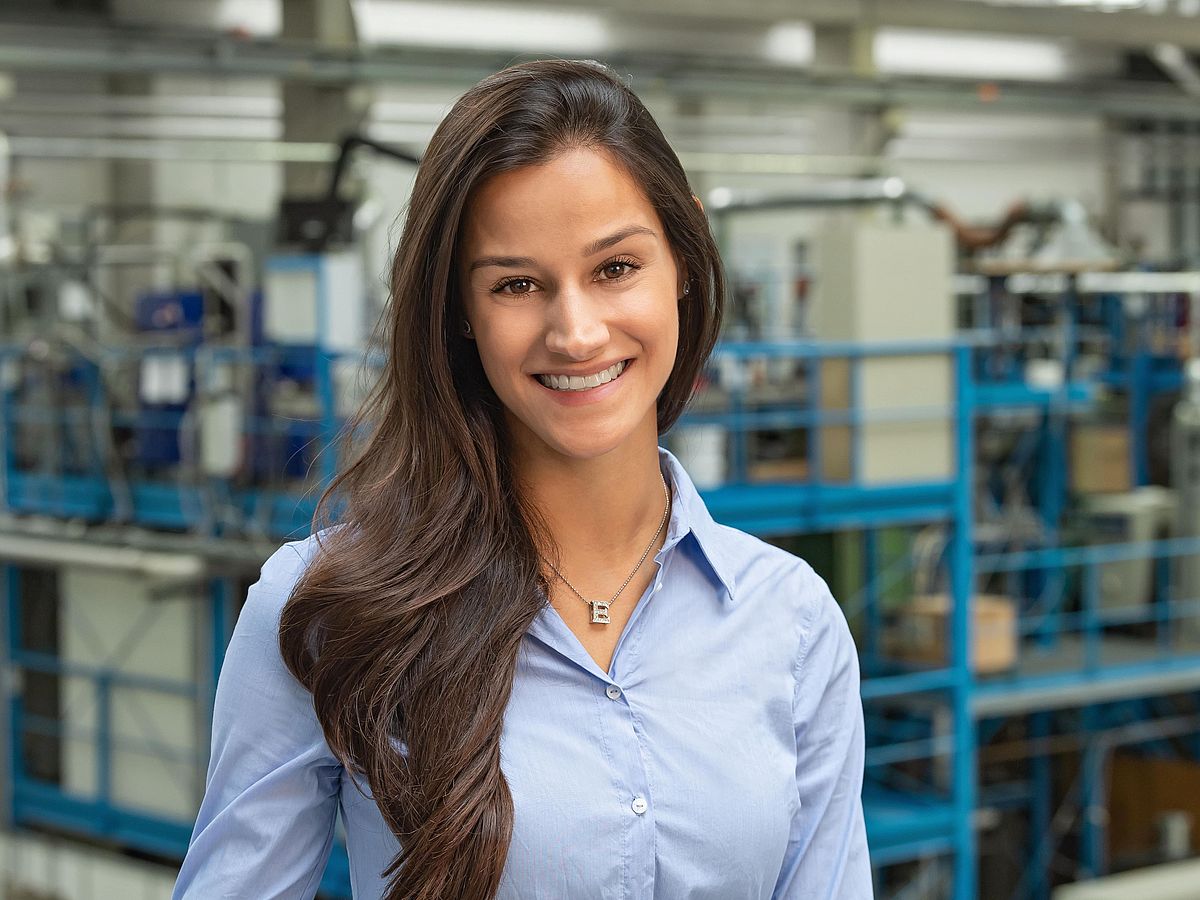
M. Sc. Erika Soares Barreto
Metallic glasses and high entropy alloys
Universität Bremen
Fachbereich 4 Produktionstechnik
Fachgebiet 01 Mechanische Verfahrenstechnik
IW1+2 1010
+49 421 64514
sbarretoprotect me ?!iwt.uni-bremenprotect me ?!.de
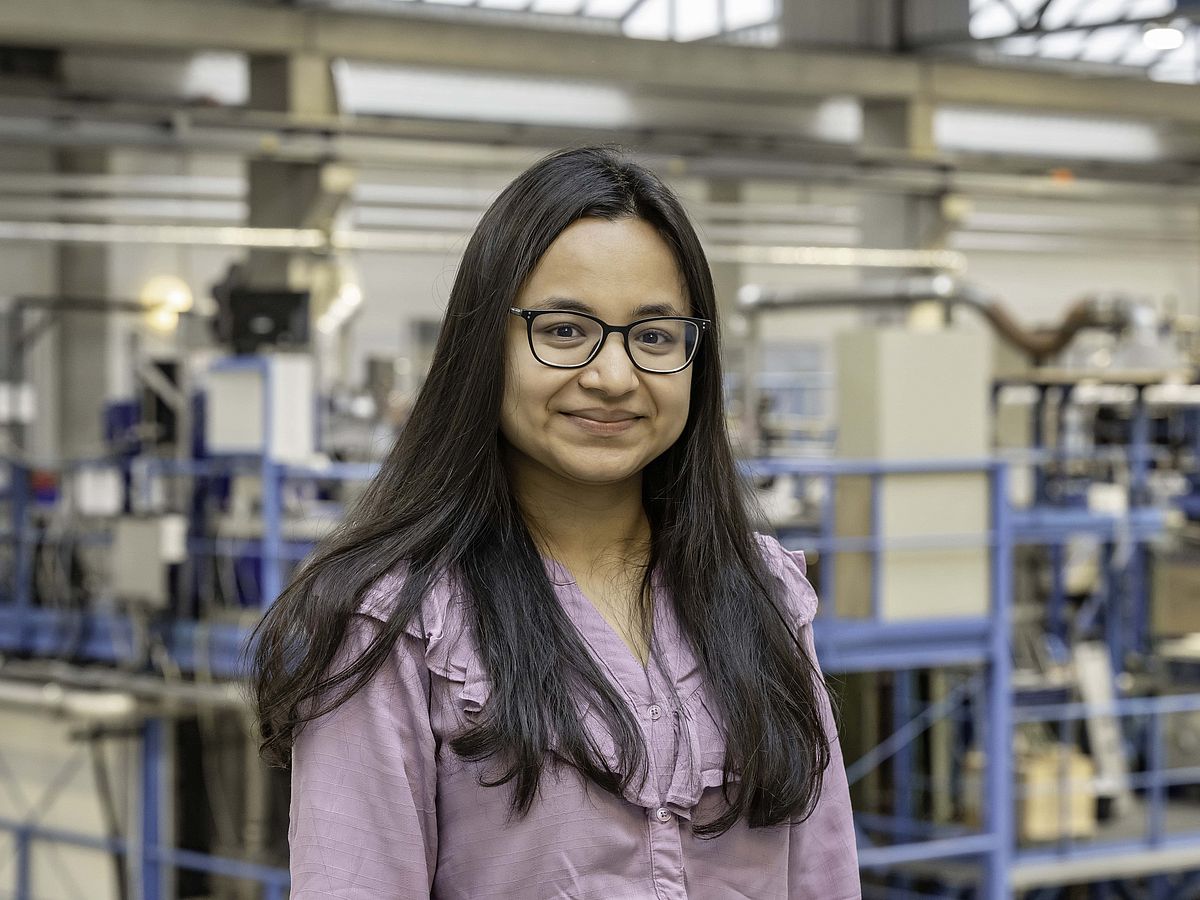
M. Sc. Layla Shams Tisha
Recycling of Al-Si-alloys through additive manufacturing
Universität Bremen
Fachbereich 4 Produktionstechnik
Fachgebiet 01 Mechanische Verfahrenstechnik
FZB 1280
+49 421 51345
tishaprotect me ?!iwt.uni-bremenprotect me ?!.de
M. Sc. Carolina Souza Santiago
Materials for H2-transport
Universität Bremen
Fachbereich 4 Produktionstechnik
Fachgebiet 01 Mechanische Verfahrenstechnik
IW1+2 1010
+49 421 64511
c.santiagoprotect me ?!iwt.uni-bremenprotect me ?!.de